There’s more to the light emitted by fusion devices than meets the eye.
Start browsing articles about fusion energy and you’ll quickly find a trove of images depicting the ethereal fuchsia glow of plasmas inside tokamaks and other fusion devices.
In reality, the pink color you see in photos comes not from fusion itself, but rather from its fuel — hydrogen — which often emits light in the specific visible wavelengths that appear pink to the human eye. Depending on its conditions, hydrogen plasma can radiate light that looks anywhere from pink to purple to blue. Or it may not emit visible light at all.
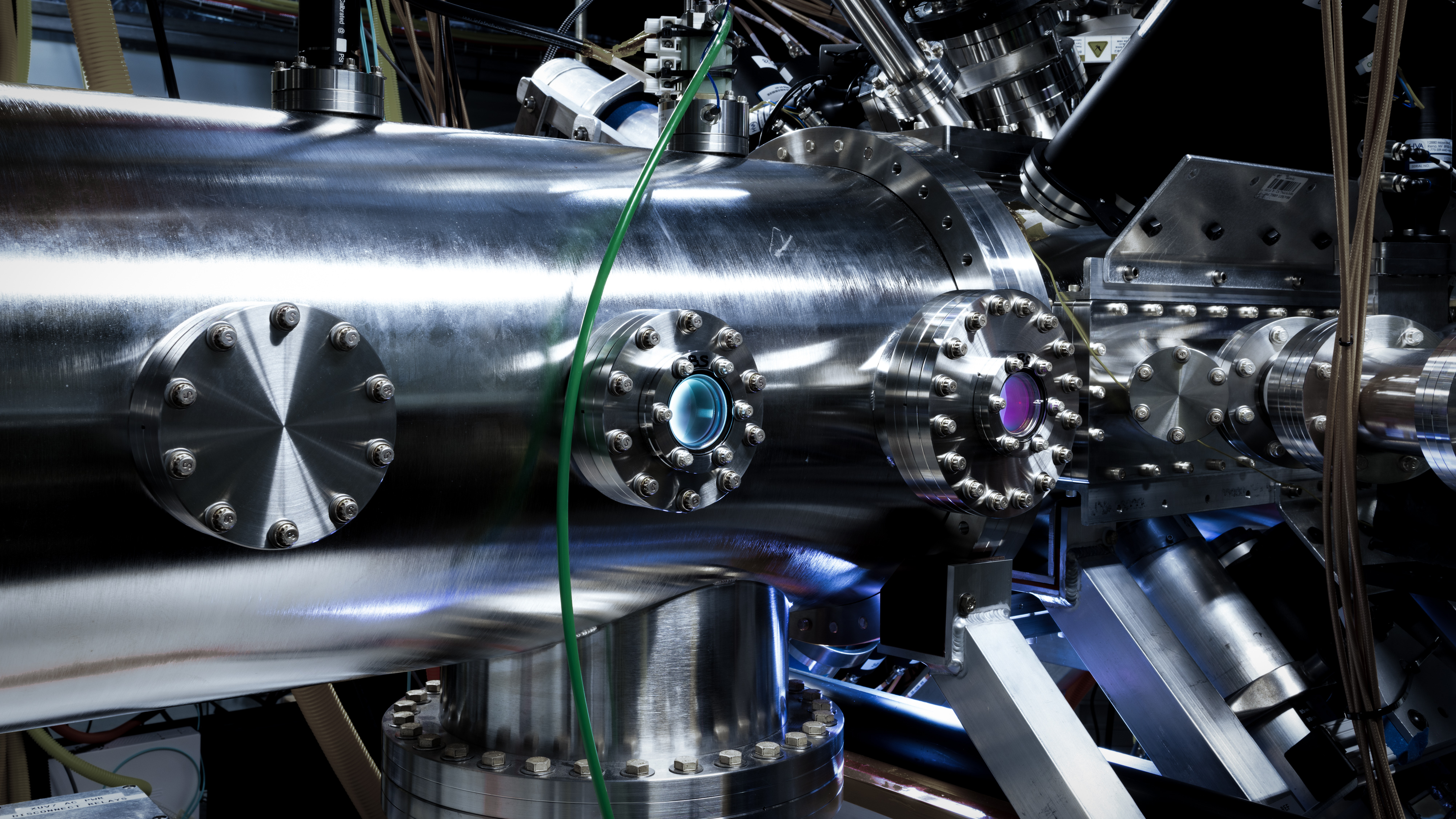
Indeed, because generating controlled fusion on earth requires extremely energetic plasmas – hotter and denser than the center of the sun – fusion plasmas radiate much of their light at energies outside of the visible part of the spectrum, such as in the ultraviolet or x-ray ranges. In other words, fusing plasma itself is so hot and energetic that it’s invisible to human eyes.
So the visible light you see in photos actually comes from cooler plasmas, often found either on the edges of hotter plasmas as they interact with colder gases, in lower power tests, or in plasmas that have already relaxed to a lower energy state.
But while you may not be seeing fusion energy generated in that pink glow, visible images can still reveal valuable details about the complex dance of plasma inside fusion devices like Zap’s FuZE and FuZE-Q. With the help of ultra-high-speed cameras, Zap scientists capture movies showing the dynamics of plasma in the chamber as fusion occurs, allowing them to benchmark against other measurements and optimize the physics.
Through the looking glass
Inside Zap’s devices, a powerful pulse of electrical current energizes deuterium gas (a natural isotope of hydrogen), and ionizes it into a plasma that can reach the superhot temperatures necessary for fusion.
Zap’s plasmas last less than a hundred microseconds (millionths of a second), so scientists use specialized, ultra-high-speed cameras to capture videos of plasma dynamics in the chamber as fusion occurs. While a standard movie camera shoots at 24 frames per second, these cameras can capture up to 10 million frames per second.
“We want to see the motion of the Z pinch. We want to see this flow that comes out,” says Dr. Andrew Taylor, a senior research scientist who oversees optical spectroscopy at Zap. “Those dynamics match our other diagnostics and give us a more holistic understanding of what's happening inside the chamber.”
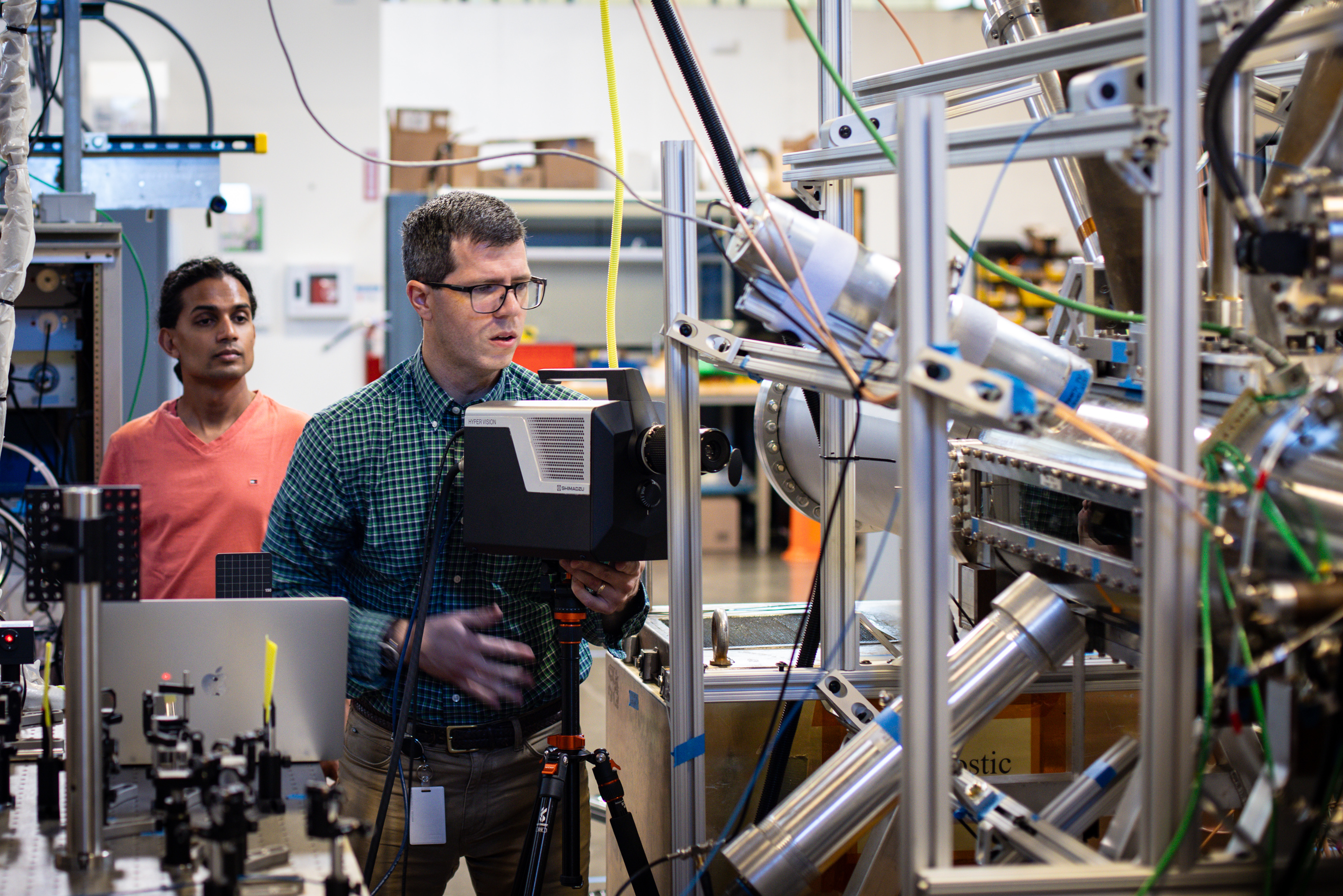
The high-speed cameras can be positioned along any one of many fused silica windows looking into the plasma chamber, or pointed down the barrel, to capture specific information about the distinct phases of Z-pinch formation: the “snowplow” of heated deuterium plasma sweeps down the chamber and assembles into the compressed Z pinch, then glowing plasma filaments form and coalesce into a moving stream. Finally, sheared flow helps stabilize the plasma. Fusion reactions occur in the hot, dense middle of that moving stream, invisible to our eyes and the cameras.
Imaging the heart of fusion formation

Techniques used to measure a plasma are collectively known as “plasma diagnostics,” and no single diagnostic tells the whole story. The National Ignition Facility, for example, has nearly 120 diagnostics available to point at its target chamber.
“For any given campaign, Zap’s R&D team may field dozens of individual diagnostics to help paint a more complete picture,” says Taylor.
Integrating a wide array of well-tested diagnostics has led to an increasingly sophisticated understanding of sheared-flow-stabilized Z-pinch plasma behavior, feeding into steadily improved computational models and FuZE device designs. Most importantly, decoding both the visible and invisible light in Zap's fusion devices ultimately helps the team increase the energy produced by a plasma, bringing us closer to a working fusion power plant.